Whereas the special theory of relativity describes the laws of motion in the ‘special’ case of objects moving in an area of space outside of the influence of a gravitational field, the general theory of relatively generalises these principles to include the effects of gravity.
General relativity is underpinned by Albert Einstein’s Field Equations, first published in 1915, which specify how the geometry of space and time is influenced by the presence of matter and radiation.
General relativity provides a refinement to Isaac Newton’s theory of universal gravitation, and accounts for several effects that cannot be explained using Newton’s theory.
Distortion of Spacetime
In the theory of relativity, time is treated mathematically as a fourth dimension. General relativity describes how a hypothetical four-dimensional grid spanning space and time – spacetime – is curved in the presence of any gravitating object, i.e. anything that posses mass or energy. This means that two gravitating objects moving through space and time will both follow paths dictated by the curvature of the spacetime surrounding them. Thus, in the theory of general relativity, gravitational attraction is considered to be due to this bending of spacetime around any object possessing mass or energy.
A simplified two-dimensional analogy of this curvature of four-dimensional spacetime is often represented using a flexible sheet of rubber stretched tight like the surface of a trampoline. If you place a spherical mass on this rubber sheet, it will be pulled downwards by the Earth‘s gravity, distorting the surface of the sheet into a curved shape in three dimensions. If a second smaller (less massive) object is then rolled along the sheet close enough to the first object, the path it takes will be determined by the curvature of the rubber sheet beneath it. This leads to the path of the second object being defected inwards towards the more massive object in a similar way that the path of an object moving through spacetime will be deflected inwards due to the gravitational influence of a larger mass.
Even the path of a ray of light will be bent by the presence of a massive object in its vicinity of space. In fact, the theory of general relatively predicts the path of light will be bent by twice the amount predicted by Newton’s theory of gravity.
This effect was first confirmed in 1919 by Sir Arthur Eddington who measured the shift in the apparent position of a star observed near to the edge of the Sun during a solar eclipse. This was due to the curvature of spacetime in the vicinity of the Sun bending the path of the distant star’s light. This observation was considered to have been the first experimental test of the theory of General Relatively.
Gravitational Lensing
In a similar way that a lens bends the path of light and can focus it to form an image, the path of light can be bent by the presence of massive objects in space. Light from distant objects, such as stars, galaxies or quasars, can be bent around closer foreground objects that lie between the light source and the observer, such as a clusters of galaxies, black holes or even dark matter. This can lead to multiple distorted images of the same background object, due to this bending of light around the foreground object that is distorting the spacetime surround itself. This effect is known as ‘gravitational lensing’.
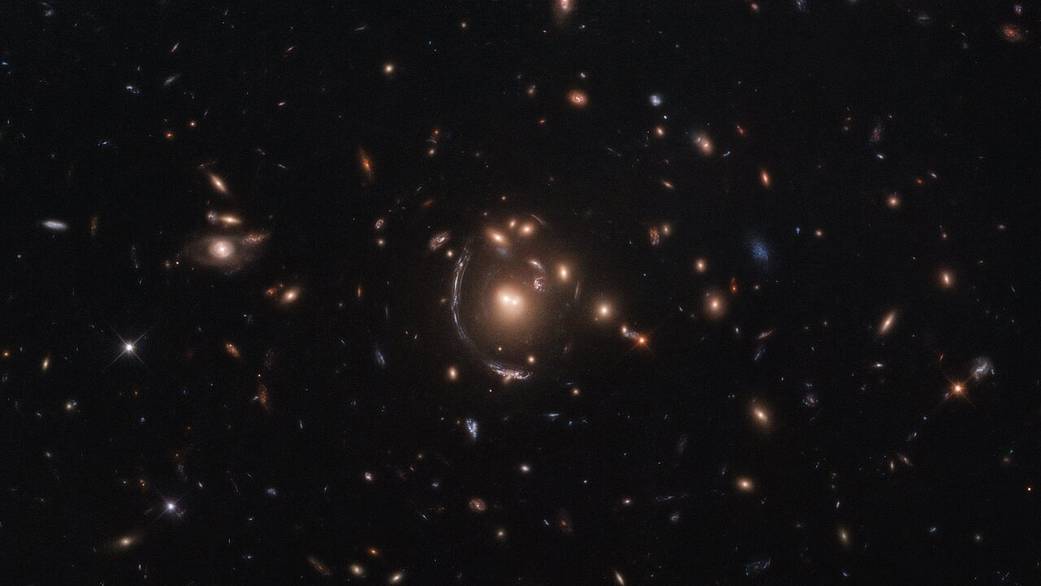
Gravitational Redshift
As a beam of light moves upwards against a gravitational field (e.g. that of a star, a planet, or a black hole) it will lose kinetic energy, which is converted into potential energy due to the increased distance from the centre of mass. Because the speed of light has to remain constant to all observers (see relativity theory), this means that the light cannot simply slow down like an object with rest mass does as it climbs upwards against gravity. This loss of kinetic energy instead translates as a decrease in the frequency of the light (from the Planck relation, where energy = Planck’s constant (h) x frequency).
This is known as the gravitational redshift, since a decrease in frequency (corresponding to an increase in the wavelength) of the light moves it closer towards the ‘red end’ of the electromagnetic spectrum. Conversely, if the light is travelling downwards, towards the centre of mass, it will gain kinetic energy and it’s wavelength will be shifted towards the ‘blue end’ of the spectrum. As the shift in frequency of the light can be in either direction, depending on which way the light is heading, this effect is often also known as the gravitational frequency shift.
Gravitational Time Dilation
This gravitational redshift means that a hypothetical light clock in sync with the frequency of a light wave in a strong gravitation field would run more slowly than a clock in a weaker gravitation field. To an observer in space watching red-shifted light from such a clock on the surface of the Earth, the clock would appear to run slower than a similar light clock aboard the observer’s own spaceship. This effect is know as gravitational time dilation.
This gravitational time dilation is not simply an optical illusion. This surprising prediction of the theory of general relativity applies to all processes that measure the passing of time, meaning that a clock placed high up on a mountain top would run faster than a clock at the bottom of the mountain. Time itself can be considered to run at different speeds at different altitudes, due to the curvature of spacetime around the gravitating object. This effect is very small, but has to be taken into account in satellite communications, for example.
If two twins were to live at different altitudes above sea level, with one going to live at the top of a mountain, then biochemical processes in their bodies that cause ageing would also take place at different rates, with the sibling who stayed to live at the bottom of the mountain ageing slower. Their biological ages would diverge, although by an imperceptible amount in this example. It should be noted, that to both twins, time would always appear to pass normally. It is only relative to the other twin’s clock that time appears to run at a different rate.
The effect of gravitation time dilation can be much more extreme in the presence of the powerful gravitation field surrounding a black hole. In fact, if you were to watch a (hypothetical indestructible) clock falling into a black hole from a safe distance, the clock would appear to slow to a complete stop as it approached the event horizon of the black hole. Because of this, any object that falls into a black hole would not appear to actually fall beyond the point of the event horizon. Instead, the object would appear to slow to a complete halt as its image is red-shifted beyond the range of visible light. To the object falling into the black hole itself, this effect would be reversed, with time outside of the black hole appearing to accelerate, as the object passes the event horizon while continuing to accelerate under gravity.
Gravitational Waves
General Relativity’s gravitational field equations predict the existence of ripples in spacetime that propagate at the speed of light. These are known as gravitational waves.
Gravitational waves were first detected by the Laser Interferometer Gravitational-wave Observatory (LIGO) in 2016, one hundred years after their existence was predicted by Albert Einstein, in 1916. The waves detected by LIGO were created by the merger of two black holes, and this new field of astronomy should provide us with new insights into such events, which cannot easily be observed through other means. See Gravitational Waves Detected.
General Relativity and Cosmology
The theory of general relativity is vital to the field of cosmology and to understanding the shape and evolution of the universe.
When Einstein first developed his field equations, he used them to try to create a mathematical model of the universe. At the time, there was no evidence to suggest that the universe is expanding, so to try to explain why the universe didn’t collapse in on itself due to its own gravity, he included an additional term in his equations that he referred to as a cosmological constant. This term described an overall curvature to spacetime throughout the universe, which would stop the universe from collapsing.
When, in 1931, it was confirmed by the observations of Edwin Hubble that the universe is expanding (see Hubble’s Law), Einstein realised that he should have predicted this from his original equations and described his addition of this cosmological constant as his “biggest blunder”. However, this concept of a cosmological constant has since been revived in an attempt to explain dark energy, which is believed to be causing the rate of expansion of the universe to increase.
General Relativity and Quantum Mechanics
Although the general theory of relativity has been tested to extremely high levels of accuracy, we know that it can’t be applied in its current form at length scales that are dominated by quantum theory, such as around the event horizon of a black hole or in the first moments following the big bang, where the universe was in an extremely dense, compact state.
In order to reconcile the two extremely successful but independently derived theories of quantum mechanics and general relativity, a new theory of quantum gravity will need to be developed. Progress on this new theory has been frustrating for theoretical physicists, who have tried unsuccessfully, so far, to create a mathematical model of quantum gravitation. Many of these theories attempt to explain gravity as a effect of the exchange of particles called gravitons, in a similar way that the other three fundamental forces of electromagnetism and the weak and strong nuclear forces are mediated by the exchange of force-carrying particles.
Candidates for this new theory of quantum gravitation, including string theory and many other approaches, are often referred to as the ‘theory of everything’ since they try to unify the concept of gravity with the other fundamental forces. Other approaches such as loop quantum gravity, attempt instead to quantise the gravitational field while keeping it separate from the other forces. Other theories such as entropic gravity, describe gravity as an emergent property and not a fundamental interaction.
To read about the special theory of relativity, visit our main page on relativity theory.